|
A Virology Primer: With Special Reference to Ozone
Gerard Sunnen, M.D.
© Copyright 1997
Revised 2005
The Nature of Viruses
Viruses are intracellular parasites. They require a living host cell in order to replicate and to infect new hosts. Viruses have been enormously successful in parasitizing all known forms of living organisms in the animal and plant worlds.
Components of Viruses
1. Nucleic acid core. At the core of viruses is genetic material
that encodes the transcription of all viral components, mostly
proteins (such as enzymes) but also lipids and glycoproteins.
This genetic material is either RNA or DNA, never both. The nucleic
acid may be single or double stranded. Viral nucleic acid has
been described as "the software program for making copies of
the virus".
2. The nucleic acid coat or capsid. Surrounding the core is a protective coating made of protein, the capsid. The capsid is rigid and determines the shape of the virus. It is made of repeating protein units called capsomeres.
The architecture of capsomere assembly is quite fascinating. A common crystal-like configuration is the 20-sided isocahedral construction, with each capsomere forming an equilateral triangle. Another pattern is helical or rod shape. The nucleic material and its capsid curl unto itself forming a spherical structure. A few viruses have capsids that are neither icosahedral nor helical. These configurations are termed complex, and may be spiral, brick shaped, or otherwise non-standard in their appearance.
The capsid and its nucleic acid core together form the nucleocapsid.
3. A membrane called an envelope, in some virions, surrounds the nucleocapsid. Enveloped viruses are usually spherical because their envelopes, unlike their capsids, are loose-fitting. Envelopes are lipid bilayers that contain phospholipids, cholesterols and proteins. Some of these proteins incorporate carbohydrates and are thus called glycoproteins. Glycoproteins usually protrude out of the envelope as spikes, the peplomers. The function of peplomers is to form points of attachments to host cell surface receptors for entry into cells.
FIGURE 1-1 SIZE OF MICROSCOPIC ENTITIES AND MICROSCOPE RESOLUTION. Viruses
are smaller than the smallest bacteria and larger than macromolecules.
They can be seen with the electron microscope. (Illustration
demonstrates relative sizes and is not drawn to scale)
From: Leland DS. Clinical Virology, Saunders, New York, 1996
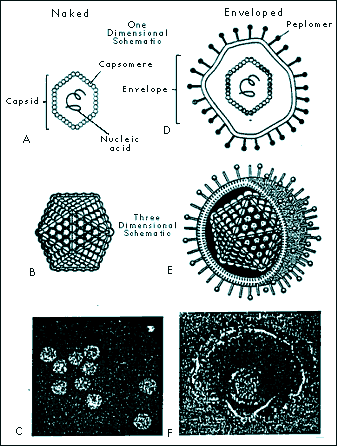
FIGURE 1-2 ICOSAHEDRAL CAPSID CONFIGURATION IN NAKED AND ENVELOPED
VIRUSES. Naked icosahedral viruses appear cubic or crystalline
(A, B, and C). Enveloped icosahedral viruses appear nearly spherical
(D, E, F). (Fig. 1-2C from Ryan KJ (Ed), Sherris Medical Microbiology:
An introduction to infectious Diseases, 3rd ed. Norwalk, CT: Appleton & Lange, 1994. Fig. 1-2F
from: Murray PR, Kobayashi GS, Pfaller MA, Rosenthal KS. Medical Microbiology, 2nd ed. St. Louis, Mosby Year Book, 1994, p 573
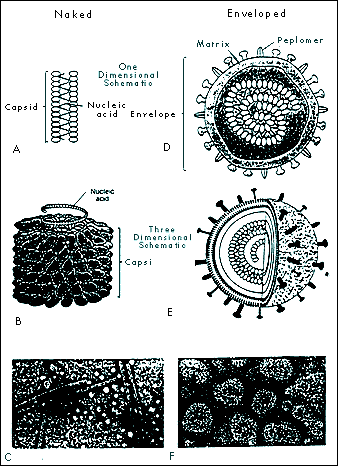
FIGURE 1-3 HELICAL CAPSID CONFIGURATION IN NAKED AND ENVELOPED
VIRUSES. Naked helical viruses are cylindrical or rod shaped (A,
B, and C). Enveloped helical viruses appear nearly spherical because
the nucleocapsid (capsid and nucleic acid) may curl inside the
envelope (D, E, and F). Fig. 1-3 C from Tortora et al. Microbiology,
4th ed. Redwood City, CA: Benjamin Cummings 1992, p 336. Fig. 1-31F
From Ryan KJ (ed), Sherris Medical Microbiology: An Introduction to Infectious Diseases, 3rd ed. Norwalk, CT: Appleton & Lange, 1994.
The envelope is sometimes connected to the nucleocapsid by a protein matrix.
4. Viruses that do not have an envelope are called "naked viruses."
5. Virions are the mature viral particle capable of infecting other cells. In non-enveloped viruses, the virion consists of the nucleocapsid alone. For enveloped viruses, the nucleocapsid and the envelope make up the virion. Enveloped viruses are only comfortable in the delicate homeostatic environment of mammalian bodily fluid milieus and are transmitted by bodily fluid transfers such as blood transfusion and sexual contact. The intestinal route, on the other hand, usually transmits naked viruses.
Enveloped Viruses Include the Following Families:
Hepadnaviridae (from Hep: liver; dna: DNA).
Hepatitis B (HBV). Long incubation (45 to 180 days). 250 million carriers worldwide or 5% of world population; 1 million in the USA. 25% develop chronic hepatitis with significant numbers evolving to liver carcinoma.
Retroviridae: (from retro: reverse, to describe the reverse transcription allowing these viruses to make DNA from RNA): HIV-1, HIV-2
Flaviviridae: Hepatitis C; West Nile virus (an Arbovirus: from Arthropod borne); tick-borne encephalitis; yellow fever; dengue
Herpesviridae: (herpes: to creep, describing creeping vesicular lesions):
Herpes simplex, types I and 2; Varicella-Zoster (chickenpox, herpes zoster); Cytomegalovirus (from cyto: cell; and megalo: large): neonatal mortality, transplantation glomerulonephritis and pneumonitis; Epstein-Barr (infectious mononucleosis, Burkitt’s lymphoma. Implicated in chronic fatigue syndrome); Herpesvirus type 6 and 7 (exanthema subitum)
Filoviridae: (filamentous viruses with hook shapes)
Ebola virus; Marburg virus
Orthomyxoviridae:
Influenza A, B, and C; Avian flu
Paramyxoviridae: (resembles Orthomyxoviridae): Measles, mumps, parainfluenza (rhinitis, laryngotracheitis, bronchiolitis), respiratory syncytial virus (flu-like syndrome, pneumonitis).
Coronaviridae: (Envelope dotted with large, club shaped peplomers resembling a crown of thorns); SARS
Togaviridae: (Toga: coat): Rubella (German measles); Eastern and Western equine encephalitis (transmitted by mosquitoes); arthritis; Rubella
Rhabdoviridae: (Rhabdos: rod): Rabies
Bunyaviridae: Encephalitis (La Crosse encephalitis); hantavirus: hemorrhagic fever with renal syndrome; hantavirus respiratory syndrome; Rift Valley Fever
Poxviridae: Large DNA viruses (smallpox; molluscum contagiosum)
Arenaviridae: Hemorrhagic fever; Lassa fever
Some Major Non-Enveloped (Naked) Viruses:
Adenoviridae: (from adenoid tissues). Adenovirus: upper and lower
respiratory infection; gastroenteritis; cystitis; pharyngoconjunctival
fever; epidemic keratoconjunctivitis
Picornaviridae: (pico: small): Enteric viruses (intestinal route
of transmission): poliovirus, hepatitis A, rhinovirus, coxsackievirus
(pharyngitis, aseptic meningitis, pericarditis, myocarditis, colds),
Echovirus (from E-enteric, C-cytopathogenic, H-human, 0-orphan):
aseptic meningitis, colds.
Rhinoviruses: colds
Papillomaviridae: Small DNA icosahedral viruses (condyloma acuminatum;
genital warts)
Caliciviridae (Calyx: cup): Norwalk gastroenteritis, hepatitis E
Astroviridae (Star-shaped virions): gastroenteritis (waterborne)
Reoviridae: Rotavirus: diarrhea in infants and adults
Novel infectious agents
In addition to enveloped and naked virions, other infectious agents
exist which defy easy classification. These are called "novel
infectious agents." Viruses need genomes to replicate.
Novel infectious agents, however, challenge the conventional
wisdom that genomes are necessary for reproduction.
Satellites are tiny RNA fragments dependent upon the enzymes of viruses for securing their progeny. Viroids, closed circular RNA molecules without capsids, do not require the presence of a helper virus. These botanical infectious agents, however, need the RNA polymerases of the cells they invade.
Prions, in humans, are responsible for Creutzfeldt-Jakob disease, familial fatal insomnia, Gerstmannaussler-Scheinker disease, and kuru. These diseases inexorably ravage the nervous system. In animals, they cause scrapie in sheep, chronic wasting disease in elk, and bovine spongiform encephalopathy, among others. Prions are transmissible infectious particles composed of quasi-facsimiles of normal body proteins. They differ, however, in that they contain only a few amino acid substitutions sufficiently minor as to make them invisible to the body’s immune surveillance.
Commentaries on the Evolution of Viruses
Viruses are far from being static entities. As quintessential intracellular parasites they have developed, through millions of years of cohabitation with their hosts, astoundingly sophisticated structures and propagation mechanisms. They have constantly modified their biological strategies, incorporating genetic diversity and mutational creativity to adapt to our ever-changing planetary ecology.
In the 21st century, this ecology, namely the human reservoir, has changed dramatically. The eruptive world population and the mobility of the planet's inhabitants are two major factors responsible for the accelerated evolution of viruses into new frontiers of pathogenicity.
Most of the families of viruses mentioned above have produced new pathogenic strains. Of great concern is the diversification of the retroviral, the hepatitis and the influenza families. In the past three decades, for example, the following viruses are among the many that have been discovered: human herpesvirus 6, 7, and 8; hepatitis C; hepatitis E; morbillivirus (encephalitis); and hantaviruses.
Viral Infection
Once viruses have penetrated into the host they are spread via the blood and the lymphatic circulations. This is called a viremia. Waves of viral particles dispatched to infect organ systems throughout the body are known as viremic episodes. Many viruses travel free in the plasma while others attach themselves to platelets, lymphocytes and red blood cells.
Research has shown that the quantity of virions involved in infection is much more important than previously realized. In hepatitis C, retroviruses, and influenza, for example, several billion new particles may be manufactured daily. Viral load is a measure of the virion population. Understandably, the immune system is placed under sustained pressure to neutralize these waves of new infective arrivals and to regenerate its own decimated cellular components.
Any reduction in the viral load by any means proffers an advantage to the immune system and thus enhances the probability for vanquishing the infection.
Ozone, a natural molecule
Ozone is a molecule composed of three oxygen atoms. Created in the upper stratosphere as a result of solar ray interactions with oxygen and thus forming the outermost layer of our biosphere, ozone possesses unique properties that are being defined and applied to clinical practice. As a molecule containing a large excess of energy, ozone, through incompletely understood mechanisms, manifests bactericidal, virucidal, and fungicidal actions that may make it a treatment of choice in certain conditions and an adjunct to treatment in others.
The oxygen atom exists in nature in several forms: (1) As a free atomic particle (0), it is highly reactive and unstable. (2) Oxygen (02), its most common and stable form, is colorless as a gas and pale blue as a liquid. (3) Ozone (03), has a molecular weight of 48, a density one and a half times that of oxygen, and contains a large excess of energy in its molecule (03 ⇒3/2 02 + 143 KJ/mole). It has a bond angle of 127° ± 3°, resonates among several hybrid forms, is distinctly blue as a gas, and dark blue as a solid. Ozone is a powerful oxidant, surpassed in this regard only by fluorine (4) 04 is a very unstable, rare, nonmagnetic pale blue gas that readily breaks down into two molecules of oxygen.
Ozone application for medicinal purposes is a specialized discipline
requiring knowledge of ozone’s physical, physiological and
antimicrobial dynamics.
The ozone inactivation of viruses
Viruses. Viruses are parasites at the genetic level, separated into families based on their structures, types of nucleic genome and modes of replication.
Recently, there has been renewed interest in the potential of ozone for viral inactivation in vivo. It has long been established that ozone neutralizes viruses in aqueous media and it stands to reason that it would be studied for similar applications in living systems. In vivo ozone applications, however, present far greater challenges. Indeed, the technology of medical ozone administration aims to respect the delicate balance of patient safety on one hand and antimicrobial efficacy on the other.
All viruses are susceptible to ozone's neutralizing action. Viruses,
however, differ in their relative susceptibility to destruction
by ozone. In one study, poliovirus resistance was 40 times that
of coxsackievirus. Relative susceptibility in ascending order was
found to be: poliovirus type 2, echovirus type 1, poliovirus type
1, coxsackievirus type B5, echovirus type 5, and coxsackievirus
type A9. In pure water, at maximal solubility of ozone and room
temperature, echovirus type 29 is inactivated in one minute, poliovirus
type 1 in two, type 3 in three, and type 2 in seven minutes (Roy
1982). Analysis of viral components showed damage to polypeptide
chains and envelope proteins, which could result in attachment
capability compromise, and breakage of the singleanded RNA
producing replicating dysfunction. Other researchers, in similar
experiments, concluded that in ozonation, it is the viral capsid
that sustains damage (Riesser 1977). Viruses, unlike mammalian
cells, have no enzymatic protection against oxidative stress.
Lipid-enveloped viruses are sensitive to treatment with ether,
organic solvents, and ozone (Bolton 1982), indicating that disruption
or loss of lipids results in impaired or destroyed infectivity.
The enveloped viruses are more fragile than the naked viruses.
Envelopes, made up of lipids, phospholipis, cholesterols and glycoproteins
are especially vulnerable to ozone's capacity for electron removal
(oxidation). Cleaving the unsaturated chemical bonds of envelope
peplomers damages the capacity of viruses for attaching to host
cells and progressing in their life cycles. In addition, once the
virion’s lipid envelope becomes fragmented, its genomic core
cannot survive.
The enveloped viruses, adapted to the delicate homeostatic milieu
of their mammalian hosts are usually more sensitive to all physico-chemical
challenges than are naked virions. Although ozone's effects upon
unsaturated complex lipids are one of its best documented biochemical
action, ozone is known to interact with other viral constituents.
This becomes relevant when ozone inactivation of non-enveloped
virions is considered.
In contrast to enveloped viruses, naked viruses constituted of a nucleic acid core and a protein capsid coating, usually show more robust resistance to chemical and oxidative challenges. This is logical, in view of their dependence upon survival in harsh environmental habitats, such as contaminated waters and fomites. Ozone, however, has the capacity to inactivate all naked viruses. Indeed, when ozone comes in contact with viral capsid proteins, protein hydroxides and protein hydroperoxides are formed, and viral demise ensues.
In summary, ozone's antiviral action in blood may recruit the
following mechanisms:
1. The denaturation of virions through direct contact with ozone. Ozone, via this mechanism, disrupts viral envelope lipids, phospholipids and lipoproteins. The presence of numerous chemical double bonds in these molecules makes them vulnerable to the oxidizing effects of ozone, which readily donates its oxygen atom and siphons electrons in redox reactions. Broken bonds are thus reconfigured, molecular architecture becomes disrupted, and breakage of the viral envelope ensues. Deprived of an envelope, virions cannot sustain nor replicate themselves.
2. Ozone proper may directly alter structures on the viral envelope that are necessary for attachment to host cells. Peplomers, the viral glycoproteins protuberances that connect to host cell receptors are likely sites of ozone action. Alteration in peplomer integrity impairs attachment to host cellular membranes foiling viral attachment and penetration.
3. Introduction of ozone into the serum portion of whole blood induces the formation of lipid and protein peroxides. While these peroxides are not toxic to the host in quantities produced by ozone therapy, they nevertheless possess oxidizing properties of their own which persist in the bloodstream for several hours. Peroxides created by ozone administration may serve to further reduce viral load by sustained anti-peplomer action.
4. Immunological effects of ozone have been documented. Cytokines
are proteins manufactured by several different types of cells that
regulate the functions of other cells. Mostly released by leucocytes,
they are important in mobilizing immune response. Ozone induces
the release of cytokines that in turn activate a spectrum of immune
cells. Ozone is reported to be an immuno-stimulant in low doses
and immuno-inhibitory at higher levels (Werkmeister 1985, Varro
1974, Zabel 1960). Additionally, ozone functions as a signaling
agent by stimulating production of nuclear factor kappa B, interleukin
6, and tumor necrosis factor æ. Ozone’s capacity for cytokine activation has been amply documented (Bocci 2005).
5. Ozone action on viral particles in infected blood yield several possible outcomes. One outcome is the modification of virions so that they remain structurally intact yet sufficiently dysfunctional as to be nonpathogenic. This attenuation of viral particle functionality through slight modifications of the viral envelope, and possibly the viral genome itself, mollifies pathogenicity and allows the host to increase the sophistication of its immune response. The creation of dysfunctional viruses by ozone offers unique therapeutic possibilities. In view of the fact that so many mutational variants exist in any one afflicted individual (e.g., hepatitis C, influenza, HIV), the creation of an antigenic spectrum of crippled and fragmented virions could provide for a unique host-specific stimulation of the immune system, thus designing what may be called a host-specific autovaccine.
6. An exciting avenue of research suggests that the virucidal properties of antibodies are predicated upon their ability to catalyse highly active forms of oxygen including ozone (Marx 2002; Wentworth 2002). In this model, activated neutrophils provided with appropriate starting materials are capable of generating singlet oxygen, a most powerful oxidant. The singlet oxygen combines with oxygen to form ozone, itself an oxidant, whose electron-extracting capacity is only second to fluorine. It can also combine with water to form the hydroxyl radical (OH) and hydrogen peroxide. Endogenously created ozone thus becomes a fundamental immunological agent for viral inactivation.
Exogenously administered ozone may, based on this model, amplify the efficacy of antigen-antibody dynamics.
Methodologies of ozone antiviral therapy
The principal and time-tested method of administering ozone for systemic viral conditions is autohemotherapy. In this technique, an aliquot of blood is withdrawn, interfaced with an oxygen/ozone gaseous mixture, and then returned to the patient. The procedure is repeated serially. The blood volume, the ozone dosage, the frequency and the total number of sessions are predicated upon the viral condition under treatment and the clinical status of the patient. In chronic conditions such as hepatitis C, for example, the treatment protocol may stretch for longer periods of time, and will adapt to the clinical course, and to the evolution of laboratory values such as liver enzymes and viral load. In infections that are marked by a sudden surge in symptoms and an explosive replication of virions, as in avian influenza, autohemotherapy may be administered much more intensively in an emergency mode.
Importantly, another, more experimental and more intensive technique of ozone administration makes use of the extracorporeal treatment of the entire blood volume using a hollow-fibre oxygenator-ozonizer (Di Paolo 2000; Bocci 2002). This approach is promising because all blood and lymphatic fluids are interfaced with oxygen/ozone gaseous mixtures thus providing direct integral anti-viral therapy. Research is needed to determine the clinical indications and the appropriate protocols for this promising ozone/blood interfacing methodology.
Ozone is an exemplary agent for neutralizing a wide range of viruses.
Medical ozone technology spares the biological integrity of host
tissues, including blood. As regards the viability of cellular
elements such as red blood cells, leucocytes and platelets, the
oxidizing potential of ozone may be accurately calibrated so that
it will be fatal to virions but innocuous to host cells.
Ozone has a propitious therapeutic range of administration. Like many other drugs, ozone may be said to optimally function within a therapeutic window defining its optimal levels of administration. Below the window, concentrations and dosages of administered ozone are poorly effective; within the window they function optimally; and above the window, toxic effects occur.
Ozone offers distinct features over other antiviral agents. It is above all, a gas. When a gas is administered to a liquid an immediate dispersion of the gas takes place thus effecting reactions in the entire fluid volume. This may be contrasted to a fluid additive, such as hydrogen peroxide, that commixes much more sluggishly with the biological fluid under treatment due to the physics of fluid-to-fluid dynamics. What is more, ozone, unlike drug therapies, is impervious to viral mutational defenses.
Viremic episodes represent sudden invasions of virions into bodily fluids. In the case of acute infections such as avian influenza, there may be one major viremic episode which, depending upon host factors, may be marked by high lethality.
In the case of chronic infections, however, such as hepatitis C or HIV, viremic episodes may occur innumerable times in periods spanning several years.
Interventions that will safely decrease the numbers of virions from the circulation will proffer an advantage to beleaguered immune functions. Viral culling via ozone hemotherapy consists in the serial treatment of aliquots of blood with precise doses of ozone. Extracorporeal ozone therapy treats the entire blood and lymphatic circulations. Judiciously decreasing viral load during viremic episodes allows for the recovery of besieged immunocompetence.
Conclusion, and Commentaries on Ozone Research
In view of these considerations, it is evident that ozone presents unique opportunities for experimental and clinical research.
Of all the antiviral agents known, none offer the special advantages ozone embodies. Ozone, because of its potent oxidizing power is able to deactivate all known viruses. It is a pan-virucidal agent, whose mechanisms of action viruses are, at this time, unable to counter.
Technologies with impeccable safety profiles have been developed to deliver ozone/oxygen mixtures to virally-afflicted patients. As a gas, ozone is incomparably able to penetrate fluids with ease and rapidity. Ozone has both immediate antiviral physico-chemical and longer-term immunological effects.
Research is compellingly needed to understand the deeper mechanisms of ozone formation in the immune system so that novel antipathogenic therapies may be discovered for responding to increasingly urgent public health needs.
Research is also needed to determine the indications for ozone administration relative to specific viral infections and to delineate disease-specific treatment protocols. Promising antiviral technologies include serial ozone hemotherapy and total blood and lymphatic system extracorporeal ozonation.
Viral diseases are expanding worldwide. This is not a science fiction scenario but a regrettable fact. Old diseases are not only finding increased population reservoirs but are also developing novel mechanisms of infectivity. Of special concern are emergent viruses, products of mutational creativity, never before encountered by humans, that are potentially devastating to health and life. Ozone embodies unparalleled promise for becoming a major contributor to the armamentarium against these new plagues.
References
Ackey D, Walton TE. Liquid-phase study of ozone inactivation of Venezuelan Equine Encephalomyelitis virus. Appl Environ Microbiol 1985; 50:882-886
Armstrong. Infectious Diseases, First Ed. Mosby, Philadelphia, 2000
Bocci V. Oxygen-Ozone Therapy: A Critical Evaluation. Kluwer Academic Publishers, Dordrecht, 2002
Bocci V. Ozone: A New Medical Drug. Springer, 2005
Bocci V, Luzzi E, Corradeschi F, Paulesu, et al. Studies on the biological effects of ozone: 5. Evaluation of immunological parameters and tolerability in normal volunteers receiving ambulatory autohaemotherapy. Biotherapy 1994; 7:83-90
Bolton DC, Zee YC, Osebold JW. The biological effects of ozone on representative members of five groups of animal viruses. Environmental Research 1982; 27:476-48
Buckley RD, Hackney JD, Clarck K, Posin C. Ozone and human blood. Archives of Environmental Health 1975; 30:40-43
Cann AJ. Principles of Molecular Virology, Second Edition. Academic Press, New York, 1997
Cardile V, et al. Effects of ozone on some biological activities of cells in vitro. Cell Biology and Toxicology 1995 Feb; 11(1): 11-21
Carpendale MT, Freeberg JK. Ozone inactivates HIV at noncytotoxic concentrations. Antiviral Research 1991; 16:281-292
Chun TW, Fauci AS. Latent reservoirs of HIV; obstacles to the eradication of virus. Proc Natl Acad Sci U.S.A. 1999; 96:10958-10961
Dailey JF. Blood. Medical Consulting Group, Arlington MA, 1998
Di Paolo N. Extracorporeal blood oxygenation and ozonation (EBOO) in man. Preliminary report. Int J Artif Organs 01 Feb 2000; 23(2): 131-141
Dixon B. Nitric oxide goes center stage. BJM 1992:305:779
Dyas A, Boughton B, Das B. Ozone killing action against bacterial and fungal species: Microbiological testing of a domestic ozone generator. J Clin Pathol (Lond) 1983; 36(10): 1102-1104
Evans AS, Kaslow RA (Eds). Viral Infections in Humans: Epidemiology and Control, Fourth Edition, Plenum, New York, 1997
Feitelson M. Hepatitis C Virus: From Laboratory to Clinic. Cambrige University Press, UK, 2002
Fleminger S et al. Prion diseases. British J Psychiatry 1997; 170:103-105
Gumulka J, Smith L. Ozonation of cholesterol. J Am Chem Soc 1983; 105(7): 1972-1979
Hansler J, Weiss H. Beitrag zum Unterschied zwischen HOT und Ozontherapie mit dem Ozonosan Erfahr hk 1976; 25:185-188
Hurst CJ. Viral Ecology. Academic Press, New York, 2000
Ishizaki K, Sawadaishi D, Miura K, Shinriki N. Effect of ozone on plasmid DNA of Escherichia coli in situ. Water Res 1987; 21(7): 823-828
Ivanova O, Bogdanov M, Kazantseva V, et al. Ozone inactivation of enteroviruses in sewage. Vopr Virusol 1983; 0(6): 693-698
Knipe DM, Howley PM. Fundamental Virology, Fourth Edition. Lippincott Williams & Wilkins, Philadelphia, 2001
Leland DS. Clinical Virology. Saunders, Philadelphia, 1996
Liang T, Hoofnagle J. Hepatitis C. Biomedical Research Reports. Academic Press, New York, 2000
Max J. Antibodies kill by producing ozone. Science 15 Nov 2002; 298: 1319
Mudd JB, Leavitt R, Ongun A, McManus T. Reaction of ozone with amino acids and proteins. Atmos Environ 1969; 3:669-682
Menzel D. Ozone: An overview of its toxicity in man and animals. Toxicol and Environ Health 1984; 13:183-204
Olinescu R, Smith TL. Free Radicals in Medicine. Nova Science Publishers, Inc. Huntington, New York, 2002
Olwin JH, Ratajczak HV, House RV. Successful treatment of herpetic infections by autohemotherapy. J Altern Complement Med 1997; 3: 155-158
Partington JR: A History of Chemistry. Macmillan and Co., New York, 1962
Paulesu L, Luzzi L, Bocci V. Studies on the biological effects of ozone: Induction of tumor necrosis factor (TNF-alpha) on human leucocytes. Lymphokine Cytokine Research 1991; 5:409-412
Prusiner SP. The prion diseases. Scientific American 1995; 272:48-57
Razumovskii SD, Zaikov GE. Ozone and Its Reactions With Organic Compounds. Elsevier, New York, 1984
Rilling S, Veibahn R. The Use of Ozone in Medicine. Haug, New York, 1987
Rice RG. Century 21 – Pregnant with ozone. Ozone Science and Engineering 2002; 24: 1-15
Riesser V, Perrich J, Silver B, McCammon J. Possible mechanism of poliovirus inactivation by ozone. In: Forum on Ozone Disinfection. Proceedings of the International Ozone Institute. Syracuse, NY, 1977:186-192
Rota P, Oberste M, Monroe S, et al. Characterization of a novel Coronavirus associated with severe acute respiratory syndrome. Sciencexpress/ HYPERLINK "http://www.sciencexpress.org" www.sciencexpress.org 2003 May 1
Roy D, Wong PK, Engelbrecht RS, Chian ES. Mechanism of enteroviral inactivation by ozone. Appl Envir Microbiol 1981; 41:718-723
Roy D, Engelbrecht RS, Chian ES: Comparative inactivation of six enteroviruses by ozone. Am Water Works Assoc J 1982; 74(12): 660-664
Sunnen G. Ozone in Medicine. Journal of Advancement in Medicine. 1988 Fall; 1(3): 159-174
Sunnen G. Possible mechanisms of viral inactivation by ozone. Townsend Letter for Doctors. Ap 1994: 336
Valentine GS, Foote CS, Greenberg A, Liebman JF (Eds). Active Oxygen in Biochemistry. Blackie Academic and Professional, London, 1995
Varro J. Die krebsbehanlung mit ozon. Erfahr hk 1974; 23: 178-181
Vaughn JM, Chen Y, Linburg K, Morales D. Inactivation of human and simian rotaviruses by ozone. Applied Environmental Microbiology 1987; 48:2218-2221
Viebahn R. The biochemical process underlying ozone therapy. OzoNachrichten 1985; 4:4:18-30
Viebahn R. The Use of Ozone in Medicine. Odrei Publishers, Iffezheim, 1999
Wells KH, Latino J, Gavalchin J, Poiesz BJ. Inactivation of human immunodeficiency virus Type 1 by ozone in vitro. Blood 1991 Oct; 78(7): 1882-1890
Wentworth P, McDunn JE, Wentworth AD, et al., Evidence for antibody-catalysed ozone formation in bacterial killing and inflammation. Science 13 Dec 2002; 298: 2195-2199
Werkmeister H. Subatmospheric 02/03 treatment of therapy-resistant wounds and ulcerations. OzoNachrichten 1985; 4: 53-59
Wolcott J, Zee YC, Osebold J. Exposure to ozone reduces influenza disease severity and alters distribution of influenza viral antigens in murine lungs. Appl Environ Microbiol 1982; 443:723-731
Yu BP. Cellular defenses against damage from reactive oxygen species. Physiological Reviews 1994 Jan; 74(1): 139-162
Zabel W. Ganzheitsbehandlung der gaschwulsterkrankungen. Hippokrates 1960; 3(1): 751-760
BACK TO MENU |